Disease and Disorders
The term plant disease refers to an impairment in the structure or function of a plant that results in observable symptoms. In this chapter the focus will be on infectious diseases—those that result from attack by a fungus, bacterium, nematode, virus, or other organisms. Other disorders can be caused by abiotic (environmental and cultural) factors, such as compacted soil, excess water, nutrient deficiencies, chemical injury, or air pollution. Many of these factors produce symptoms similar to those caused by infectious agents. Some detective work is often necessary to figure out what is wrong with a particular plant. This chapter provides an introduction to the causes of plant diseases, their diagnosis, and the methods used to prevent and control them. For information on particular diseases, refer to the chapters on specific types of plants.
Healthy and Unhealthy Plants
To recognize a plant problem, you must first know what a healthy plant looks like. Although this may seem obvious, some plants have characteristics or habits at certain stages of growth that can be mistaken for symptoms of the disease. For instance, patterns of light and dark colors on foliage can sometimes indicate disease, but color patterns can also be normal variegation in certain varieties. Sunburst honey locust might appear to be suffering from a nutrient deficiency because it has yellow-green leaves, but this coloring is normal for this cultivar. The spore-bearing sori on the underside of a fern frond might be mistaken for insects. The male cones on the tips of arborvitae foliage look somewhat like galls. Leaves on some evergreen trees (such as some hollies and magnolias) drop in the spring as new leaves expand; homeowners new to North Carolina might become concerned if they expect leaves to shed only in the fall. None of these examples are diseases. Instead, all are normal plant structures and responses.
In order to talk about diseases and disorders, we need a set of terms to describe plant abnormalities and pathogen structures.
Symptoms are not unique to a particular disease as a specific symptom can be caused by a variety of pathogens.
Chlorosis (adj. chlorotic): Yellowing of a normally green plant part. If it is more pronounced between veins it is called “interveinal chlorosis.” |
Necrosis (adj. necrotic): Death of plant tissue. A necrotic leaf blotch is pictured. Note that any well-defined dead area of a leaf, stem, or root can be called a “necrotic lesion.” |
Example of necrotic (dead) leaf spots with chlorotic (yellow) halos |
Shot-hole: Clean-edged, round to oval holes in leaves where necrotic spots have fallen out . |
Wilting: Loss of turgor in all or part of a shoot. |
Scorch: Necrosis and desiccation of leaf tissue, starting at the margins. |
Mosaic or mottle: Patchwork of colors, usually light-green, yellow, or dark-green, against the normal green background color of the leaf. |
Ringspot: Chlorotic or necrotic rings or arcs surrounding healthy tissue. |
Water-soaking: A dark, “wet” appearance to a spot, best seen by holding the leaf up to a light source. |
Flecking or stippling: Numerous very small chlorotic or necrotic points. |
Blight: Extensive and rapid death of plant tissue. |
Dieback: Death of a branch from the tip down. |
Canker: Sharply-defined dead area on a woody plant part. |
Example of a canker visible only under the bark. |
Root rot: Decay of roots. The exterior portion is easily pulled off the central core of vascular tissue. |
Fruit rot: Decay of fruit. May be firm or soft. |
Mummy: A dried, shriveled fruit. |
Damping-off: Death of seedlings, before or after emergence from the soil. |
Malformation: Any deviation from the normal shape of a plant organ. |
Leaf galls: Swellings on leafy tissue. |
Stem galls: Swellings, usually woody, on stems. |
Root galls: Swellings on root tissue. |
Gummosis: Exudation of sticky sap. In conifers, this is known as “resinosis.” |
Witch’s broom: Abnormal proliferation of shoots on one area of a stem. |
Vascular discoloration: Darkening of the plant’s conductive tissue. Visible after cutting along or into the stem. |
Specific terms are used to describe the overall patterns of death or discoloration in turf, independent of what the symptoms may be on individual leaves, stolons, and other parts. These are known as stand symptoms. Several of the most important are illustrated below. All photos are courtesy of NC State University.
Spots: Each area of affected turf is less than 4” in diameter. |
Patches: Irregularly shaped areas greater than 4” in diameter. |
Circle: Perfectly circular areas greater than 4” in diameter. |
Rings: Surrounded by healthy turf to the inside and outside of the affected area. |
Irregular: No visible pattern. |
|
Here we see the causal organism itself, usually a fungus.
Mycelium: The visible vegetative body of a fungus, made up of threads called hyphae. (sing., hypha). |
Gray mold): Fungal surface growth, gray in color. |
Powdery mildew: White surface growth on living leaves, stems, flowers, or fruit. With time this can turn gray or develop minute black flecks within. |
Downy mildew: Group of diseases characterized by white, bluish, or gray sporulation on the underside of leaves. |
Sclerotium (pl. sclerotia): Hardened brown or black fungal survival structure. May be round or irregular. |
Rust: One of a group of important fungal diseases with multiple stages, at least one of which typically produces a dry yellow to orange spores. |
Rust (see above). Example of the aecia of quince rust on ornamental pear fruit. Note the tubular white membranes that cover the spore-producing pustules in this species. |
Rust (see above). Example of the gelatinous telia of cedar-apple rust. |
Fruiting body: Any spore-producing structure of a fungus. Many are small and dark. Some grow on the surface of leaves or stems. |
Canker: Fruiting bodies that develop in a canker, below the bark, and break through the surface. |
Mushroom: A soft, stalked fungal fruiting body. One genus —Armillaria—is a plant pathogen. Most are mycorrhizal or are secondary decomposers. |
Conk: A tough, often shelf-like fungal fruiting body emerging from standing, fallen, or buried wood. Sign of wood decay in progress. |
Dodder: A parasitic plant with yellow to orange stringlike stems and inconspicuous flowers. |
Slime mold: A group of soil-, litter-, or bark-dwelling organisms, usually unnoticed until the conspicuous spore-producing phase develops. |
Abiotic Disorders of Plants
When the cause of a problem is an environmental condition, cultural practice, or chemical exposure, the causal agent is abiotic (nonliving). Disorders caused by abiotic factors are not contagious, but such disorders can severely damage plants. In these cases, it is more appropriate to use the term injury or disorder rather than disease. Most environmental problems are caused by deficiencies or excesses of factors that support life (including soil moisture, light, and temperature). Simple actions such as soil testing, finding out if a plant prefers sun or shade, loosening the edges of the root ball when planting, watering during dry weather, mulching, and knowing when to lime, fertilize, and prune are major factors in preventing many plant problems. Symptoms such as leaf yellowing, poor vigor, and dieback are the plant’s signal something is wrong with its environment.
Moisture
Both excess moisture and lack of moisture can damage plants. Extreme waterlogging results in root death because of reduced oxygen levels in the root zone. More commonly, excess soil moisture is a contributing factor to root disease. Another disorder that sometimes occurs under high moisture conditions is edema, which appears as numerous swollen bumps on the lower side of leaves. These swellings later turn brown and corky. Edema is common on certain thick-leaved herbaceous and woody plants such as geraniums, camellia, and euonymus. At the other extreme, insufficient moisture can result in scorch symptoms on foliage, stunting, leaf yellowing, leaf drop, and abortion of flowers and fruits. Necrosis can occur on the tips, margins, and interveinal regions of leaves, for example, on sycamore and dogwood trees. Under certain circumstances, potting mixes, mulches, or soils can become hydrophobic (water-repellent). Rain and irrigation will not be effective at getting water to the roots once a hydrophobic condition has occurred. Another type of moisture problem is winter burn. Roots cannot extract water from frozen soil, so the foliage of evergreen trees and shrubs can get a scorched appearance when winter winds dry them out.
Plant Nutrition
Excessive fertilization can result in root burn and plant damage from high concentrations of soluble salts. Lower-than-optimum levels of nutrients usually result in diminished growth and a wide variety of foliar symptoms, depending on the nutrients involved. Even when nutrients are present in the soil, they may be unavailable to plants if the soil pH is not in the correct range. One common nutritional problem that can be mistaken for a disease is the blossom end rot of tomatoes and peppers. Flattened, tan-colored dead spots appear on the fruit, around the point where the flower was attached. This often happens on the first cluster produced on a given tomato plant. In peppers, the damage can also appear elsewhere on the fruit. The affected areas may become dark if secondary molds develop in the affected tissue. The cause is a localized calcium deficiency in the developing fruit. It can be brought on by water stress or by low levels of calcium in the soil. High levels of fertilizer salts—especially ammonium nitrogen—can also contribute to the disorder.
Light, Temperature, Wind, and Weather
Some plants require shade. Sensitive plants such as aucuba can show burn on those leaves most exposed to the sun. Fleshy vegetables such as peppers and tomatoes can show sunscald on fruit. If a large tree is removed from a landscape, the sudden increase in sunlight can damage previously shaded plants. Other plants require sunlight. When planted in shade they have spindly growth until they reach adequate light.
Cold can damage plants that are grown out of their area of adaptation or plants exposed to rapid drops in temperature. Tropical indoor plants are prone to injury from low temperatures—both in the home and while in transport. This kind of chilling injury usually shows up as a blackening of plant tissues soon after the exposure.
Perennial and woody plants develop some tolerance to cold as fall and winter progress but lose this hardiness with the onset of spring. In the fall, cold hardiness first occurs in the terminal buds. The last tissues to go dormant are at the base of the main stem. An early freeze in the fall or a late freeze in the spring results in bark splitting or loss in those lower stems. The plant may not show distress until the heat of summer when the damaged stems are unable to move sufficient water to the foliage. Late frosts often damage the flower buds of peaches, cherries, apricots, and strawberries. Frost damage to new conifer needles will uniformly kill all needles of the same age back to the same point.
Dry winds can pull moisture from leaves, resulting in a scorched appearance. Heavy winds can remove leaves and limbs and effectively sandblast plants. Ice storms can result in broken limbs. Hail can punch holes in leaves or knock plants down. In addition, lightning strikes, fire, and high temperatures can damage or even kill a plant.
All of the abiotic plant problems described above occur naturally. Several other abiotic problems are caused by humans. Examples include air pollution, herbicide injury, and mechanical damage (for example, when a lawnmower hits a tree). Other human-caused abiotic problems include roots girdling on plants left too long in a small pot and compacted soil.
All of these abiotic injuries may attract insects and become entry points for diseases.
Plant Pathogens
We can classify diseases according to the symptoms they cause on particular plant parts. For example, there are leaf spots, fruit rots, petal blights, and other diseases named after plant parts. As a general rule, leaf spot diseases are the least serious because the plant can compensate. Exceptions occur when leaves are killed quickly, as with late blight, or when leaves drop off in large numbers, as with black spot of rose or boxwood blight. Canker diseases on woody plants are more serious because entire limbs can sometimes be affected. Root and crown rots are even more serious because a compromised root system will weaken or even kill entire plants. To make matters worse, the soil inhabitants that attack roots tend to persist from year to year. Perhaps the most destructive group of plant diseases are vascular wilts such as Dutch elm disease or fusarium wilts. In these cases, the water-conducting vessels of the plant are impaired, and decline is rapid and irreversible. One of a gardener’s challenges is to identify whether the disease will affect a plant’s long-term health and if not, whether management is necessary.
An alternate way to classify plant diseases is according to their cause. We use the term pathogen to refer to any organism that can cause disease. Some pathogens affect only a single kind (genus) of plants. Others can infect many members of a single plant family, and still, others have an extensive host range and can attack plants across multiple families. Examples of pathogens include fungi, bacteria (including phytoplasmas), nematodes, viruses, and parasitic plants.
Fungi
Fungi are a diverse group of organisms that include everything from mushrooms to molds. In most cases, the fungal “body” consists of fine microscopic threads that grow and branch out through substrates, such as soil, plant parts, foods, or even skin (in the case of the athletes’ foot fungus). There are almost 100,000 described fungal species. Estimates of the total number of fungi on earth range upwards of 5 million. Fungi lack chlorophyll and thus cannot manufacture their own food. Instead they “feed” by using enzymes to break down organic compounds produced by plants and animals.
Although some fungi are plant pathogens, the vast majority are harmless or even beneficial. For example, saprobic (also known as saprophytic) fungi obtain nourishment from dead plants and animals. In the process, they decay wood, leaf litter, and other debris. This is a part of the earth’s nutrient cycle—a beneficial service unless the wood happens to be part of your home! Other important functions of fungi include flavoring some cheeses, causing the bread to rise, fermenting beer and wine, and producing certain antibiotic precursors.
Examples of common fungal foliar diseases are black spots of rose, powdery mildew, downy mildew, brown patch, and oak leaf blister. Botryosphaeria andPhomopsis species are fungi that commonly cause cankers in woody plant parts. More serious canker diseases include chestnut blight and thousand cankers disease of walnut. Pythium, Phytophthora, and Armillaria species are important causes of root rots. Dutch elm disease and fusarium wilt, already mentioned above, are caused by fungi, as are verticillium wilt, laurel wilt, and oak wilt. Abnormal growth incited by fungi can include twisting and curling of leaves, stunting, or galling, as in the case of black knot or cedar-apple rust.
The life of a fungus may include some time on a plant, in the soil, or on plant debris. Survival and growth of the fungus depend on favorable temperatures and moisture levels. Fungi can be spread by one or more of the following: wind, water, insects, soil, or people. A few have more exotic means of transport, such as via birds or on seeds. Figure 5–56 shows the life cycle of the fungus that causes brown rot on peaches.
The microscopic threads that make up the fungal body are called hyphae, a term that comes from the Greek word for “web.” A visible mass of hyphae is called amycelium. At some stage in their development, the hyphae of many fungi group together to produce other visible signs, such as powdery mildew, conks, or mushrooms. Table 5–2 illustrates a number of these fungal signs.
Some fungi, such as Rhizoctonia solani, spread mainly by the growth of hyphae. But most fungi reproduce via spores. Spores are formed either directly on specialized hyphae or within a fruiting body of some kind. Spore shapes and spore-bearing structures are unique to particular species and can be used to identify fungi under a microscope. Fungal spores almost always require free (liquid) water for a period of time before they can germinate. If foliage stays wet for a certain number of hours at a sufficiently warm temperature, spores of fungal pathogens will germinate, forming hyphae that grow into the plant. Spores that land on dry plant tissue may lie there for several days until moisture becomes available. Depending on the particular fungus, entry into plants can be directly through the cuticle, through stomata, or via pruning cuts, leaf scars, or other wounds.
Some fungal spores are thick-walled and can tolerate dry or cold conditions, but some fungi survive these hardships by forming sclerotia. These small, hard bodies survive in plant tissue or in the soil. Examples include the sclerotia of the fungus Sclerotium rolfsii, which resemble radish seeds in size and color, and those of Sclerotinia sclerotiorium which are black, irregular, and look rather like mouse droppings.
Bacteria
Bacteria are microscopic, single-celled organisms with no organized nucleus. They live in incredibly diverse environments where they have many ecological roles. Plant pathogenic bacterial species number in the hundreds (versus thousands for fungi). Because bacteria look a lot alike under the microscope, they are usually identified by isolating them from plant tissue. Then differences in colony characteristics, biochemical properties, and DNA are used to make the identification.
Unlike many fungi, bacteria cannot penetrate plant cuticles. Rather, they enter through wounds (either caused by human activity or by insect feeding) or through natural openings such as lenticels, hydathodes, and stomata. Bacteria can be spread from plant to plant by way of soil, insects, splashing water, infected seeds, or pruning tools. The bacterium that causes fire blight can be spread by bees. Bacteria do not have specialized survival structures, so many will not survive in infected plant debris once it decays. Unfortunately, some bacteria can survive long-term in the soil or in cankers of woody plants.
Bacteria can cause leaf spots, blights, cankers, and wilts, as well as fruit, stem, and crown rots. Many leaf spots caused by bacteria are angular or linear and have straight edges because spots expand easily between but not across leaf veins. They may also have a water-soaked appearance when held up to the light. With some bacterial leaf spots, a yellow halo surrounds the lesion. Bacterial rots often lead to a slimy texture and a foul odor. How much a disease develops and how quickly it spreads depends on temperature, humidity, maturity of the plant tissue, and susceptibility of the plant. Although there can be distinctive characteristics of bacterial disease, it can sometimes be difficult to distinguish bacterial diseases from those caused by fungi. For example, bacterial leaf spot of English ivy looks a lot like anthracnose, and Alternaria leaf spot of zinnia can be mistaken for bacterial leaf spot of that same host.
The bacterium that causes the southern bacterial wilt of tomatoes and several other plants is soilborne. The bacteria enter the roots and multiply in the xylem tissue. As these water-conducting channels become clogged, plants wilt and die.
Phytoplasmas are an unusual group of bacteria that lack cell walls. They inhabit the phloem of plants and have been associated with several plant diseases. The best known of these is aster yellows, a disease that can infect many other host species besides asters. The aster yellows phytoplasma are spread by leafhoppers. The phytoplasma cause symptoms that include yellowing of foliage, the abnormal green color of flower petals, and shoot proliferation. Xylella fastidiosais another unusual sort of bacterium. It causes the bacterial scorch diseases of sycamore and oak, and Pierce’s disease of grapes. While not a phytoplasma, Xylella fastidiosa is likewise spread by leafhoppers.
What’s in a Name?
Some disease names are confusing simply because the names resemble one another. Be careful to explain that sooty mold is a fungal growth on insect honeydew (easily rubbed off), while sooty blotch is a fungal infection of the surface layer of apple fruit. Likewise, slime mold refers to a group of harmless organisms living in mulch or soil, while slime flux is the discharge of a fermenting liquid from trunks of hardwood trees in midsummer.
At other times confusion can arise when a single name is used in different ways. There’s no problem with “black root rot,” a disease of plants such as carrot, pansy, Japanese holly, and many herbaceous perennials, caused by the fungus Thielaviopsis basicola. Drop the middle word, though, and you have “black rot,” a term used for a disease of crucifers caused by the bacterium Xanthomonas, a disease of grape caused by the fungus Phyllosticta, and a disease of sweetpotato caused by the fungus Ceratocystis. Another example, black leg of geranium, is caused by the water mold Pythium, while black leg of crucifers is caused by the fungus Phoma.
The use of scientific names of pathogens can help avoid some of these problems, but sadly there is confusion in the ranks regarding scientific names, too. Some fungi have traditionally borne more than one name, depending on whether the sexual or asexual form is observed. Scientists are in the process of sorting this out, so stay tuned.
|
Nematodes
Nematodes are tiny roundworms. Some are famous for causing human and animal diseases such as hookworm, river blindness, and heartworm. Others are beneficial, for example, those used to control fungus gnats in greenhouses. Several hundred species of nematodes—all of them microscopic—are parasites of plants. Most of these are soil inhabitants and feed on plant roots. Nematodes can cause economic losses on everything from turfgrass to peach trees. The host range of individual nematode species can be fairly narrow or tremendously wide. Some nematodes remain outside the root while feeding, while others actually enter it. Once inside the root, some types migrate, while others become sedentary at a particular feeding site. All plant-parasitic nematodes have a needlelike mouth structure called a stylet. The stylet is used to puncture plant cells so that the nematode can obtain food or inject substances into the plant. The direct damage caused by nematodes is not only harmful, the damage also can predispose the host to other kinds of diseases.
Nematode damage interferes with root uptake of water and nutrients. This is why the above-ground symptoms resemble those of other stresses. Nematode symptoms include poor growth, small leaves, wilting, and off-color foliage. Examination of the roots may reveal stunted root systems, dark lesions on roots, or galls. The root-knot nematodes (genus Meloidogyne) are the most destructive plant-parasitic nematodes in North Carolina, in part due to their wide host range. They inject growth-regulating substances into root cells that stimulate the formation of galls or knots that are visible to the unaided eye. The size of the gall varies with the host. The adult nematodes themselves remain hidden within the gall. These galls should not be confused with the nitrogen-fixing nodules formed on the roots of legumes or with the normal swellings on the roots of plants such as liriope, mondo grass, and daylily.
The amount of damage caused by nematodes depends on four factors:
1. The particular species of nematodes present
2. The population levels of the nematodes
3. The particular species of plant involved
4. Growing conditions, such as moisture levels and nutrient status
The plant-nematode combination is critical. For example, we worry about the spiral, lesion, and root-knot nematodes on boxwoods. But our major nematode concern for azaleas is the stunt nematode.
All nematodes reproduce by eggs. Juveniles hatch from the eggs and develop into adults after a series of molts. Many nematode species can survive and complete their life cycles on weeds. In addition, many nematode species are able to survive long periods even in the absence of a plant host. Egg masses are an important overwintering stage for root-knot nematodes, and the eggs of cyst nematodes are protected in a sort of shell formed from the dead body of the female that produced the eggs. These cysts are just barely large enough to be visible on the surface of the roots if you look closely. Fortunately, we seldom see problems with cyst nematodes in North Carolina, except on soybeans.
On their own, nematodes can travel horizontally in the soil only inches to a foot or so per year. For long-distance spread, nematodes rely on the movement of soil clinging to boots, tools, or equipment, and on the shipment of transplants. Once introduced into a new site, it may take years for populations to build to the point where plant injury is noticed.
If you suspect that your soil has plantparasitic nematodes, submit a sample to your county’s Extension center or to the NC Department of Agriculture & Consumer Services for testing.
Two important kinds of nematodes do not fit the general pattern described. Foliar nematodes directly enter leaf tissue, where they cause yellowing and then necrosis. As with bacterial leaf spots, these affected areas tend to be limited by the veins in the leaf and so are angular (as for example in butterfly bush) or linear (as in the case of hosta). Foliar nematodes are particularly a problem in greenhouses and nurseries. The pine wilt nematode is destructive to non-native trees in the pine family. The pine wilt nematode lives within the wood of the trees and is transmitted by a type of longhorn beetle. In North Carolina, the greatest problem with pine wilt nematode is on Japanese black pine along the coast.
Viruses
Plant viruses are very small particles of either DNA or RNA wrapped in a protein coat. They can be visualized only with the aid of powerful electron microscopes. Approximately a thousand plant viruses have been described, with more being discovered continually. Viruses enter plant cells and multiply by using the plant’s own enzymes to manufacture more virus particles. Damage to plant cells occurs because normal cellular processes are disrupted.
Viruses are diagnosed by using knowledge of the viruses affecting certain hosts, observing plant symptoms, and conducting laboratory tests. Symptoms of virus infection are extremely diverse. They can include green and yellow mottling or mosaic, ring spots, color break on flowers, and sometimes necrotic spots. With some viruses, vein clearing (veins are lighter than normal) or vein banding (leaf tissue alongside veins is a darker green than the rest) develops. Stunting leaves, flowers, and entire plants is not uncommon. With a few viruses, leaf malformation or shoot proliferation (witch’s broom) can occur.
Care must be taken when interpreting some symptoms. Chimeras—genetic aberrations in chlorophyll production—look at first glance like a virus-induced mosaic. A “shoestring” distortion of tomato leaves can be caused both by Cucumber mosaic virus (CMV) and by exposure to synthetic auxin herbicides. Witches broom in rose can be caused by Rose rosette virus or by exposure to the herbicide glyphosate. Care should also be taken when interpreting virus names. Rose rosette virus infects only roses, but Cucumber mosaic virus has hundreds of hosts.
Viral infections of plants are incurable and also systemic, meaning that the virus occurs throughout the plant. Any plant that is vegetatively propagated from a virus-infected mother plant will also be infected. This includes propagation by division, cuttings, air-layering, tubers, and grafting. On the other hand, relatively few viruses are transmitted by true seed and very few via pollen. A handful of extremely hardy viruses can be “mechanically” transmitted on hands or tools. These include the Tobacco mosaic virus and its relatives, as well as the Hosta virus X. Many viruses are transmitted by arthropods, such as aphids, thrips, whiteflies, leafhoppers, and eriophyid mites. These insects and mites are referred to as vectors of the virus. For example, thrips are the vector of Tomato spotted wilt virus. So-called “soilborne” viruses are actually spread by nematodes and fungi in the soil. Most viruses die quickly outside a living cell, but they can overwinter in perennial or woody plants and in weeds.
Viroids are virus-like particles consisting of RNA but with no outer protein coat. They cause diseases similar to those caused by viruses and are spread mechanically or via propagation.
Rose Rosette Disease
It is not easy to determine whether a rose bush has rose rosette disease (RRD), which is caused by the Rose rosette virus. Symptoms vary among different rose cultivars, and can change as the disease progresses. Excessive (hyper-) thorniness is a sure sign of RRD, but thorniness does not always occur. Abnormal elongation of shoots, with a retention of the juvenile red coloration (Figure 5–64) in the mature leaves, is a strong indicator of RRD, but it can be hard to be sure you are not looking at normal coloration, especially when presented a single cane. Shoot proliferation (witch’s broom) can occur with RRD, but this symptom can also be caused by exposure to low doses of the herbicide glyphosate. A bright yellow mosaic without leaf deformation represents a much less serious disease called rose mosaic. Because laboratory confirmation of RRD is time-consuming, diagnosticians at the PDIC usually rely on an array of symptoms and the presence of the microscopic eriophyid mite vector when making a diagnosis. Familiarize yourself with the Pest News article (Volume 27, number 21, page 10-12) on this disease, but be prepared to say: “I’m not sure. Let me get a second opinion.”
|
Parasitic Plants
Parasitic plants include dodder, mistletoe, witchweed, and broomrape. Parasitic plants derive nourishment from the host plant’s vascular system. Dodder and mistletoe attach themselves to aboveground portions of a plant, whereas witchweed and broomrape attach themselves to plant roots. Dodder produces twining yellow to orange stems that resemble spaghetti. Witchweed is a serious pest of corn and has been the subject of quarantine efforts over the last several decades. Native to Africa and Asia
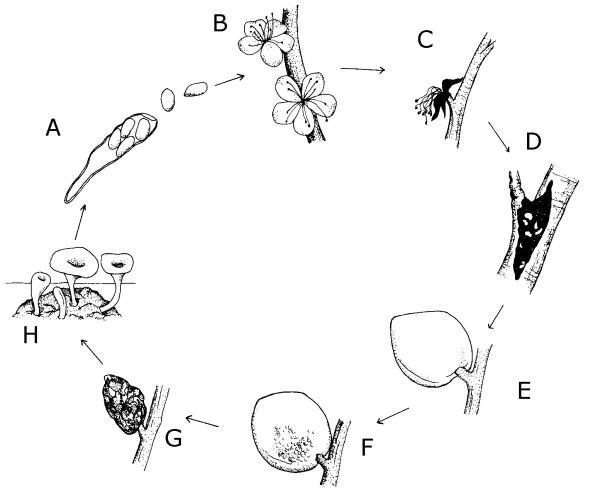
Figure 5–56. The life cycle of Monilinia fructicola A. Spores in the wind, B. Spores infect blossom, C. Blossoms killed, D. Canker develops, E. Fruit infected by spores, F. Fruit rots during summer, G. Fruit mummifies, H. Fruit produces spores early spring.
Disease Development
Although we consider pathogens as the causes of plant diseases, the development of a disease is not automatic. It also depends on the plant and the environment. Each species of plant is capable of being infected by only certain pathogens. A plant is considered susceptible when a pathogen can cause infection. If a pathogen cannot infect a plant, even under conditions favorable for disease development, the plant is considered resistant to that pathogen. Plants have varying degrees of resistance, which can change with each plant’s growth stage. For example, brown rot will develop only on maturing fruit, but peach scab develops on younger fruit. The virulence (aggressiveness) of pathogens can also vary. The environment, especially temperature and the presence or absence of water, will determine how much disease develops. By a long tradition, this relationship is depicted as the disease triangle. Because the process requires time—usually days to weeks—the time element is also included in the diagram. In some versions, the influence of a vector or of human activity is depicted as a fourth corner, making it a disease pyramid.
The beauty of the disease triangle is that it provides a framework for disease management strategies. You can grasp it at one or more of the corners. For example, modifying the environment is one method of controlling disease development. The goal is to make the environment unfavorable for the pathogen, favorable for the plant, or both. You can adjust planting dates, change the spacing between plants, provide good drainage, and avoid wetting leaves during irrigation. Using resistant plants is another strategy, based on the next corner of the triangle. Addressing the pathogen can be key, for example, because some diseases occur only during certain well-defined periods of the year.
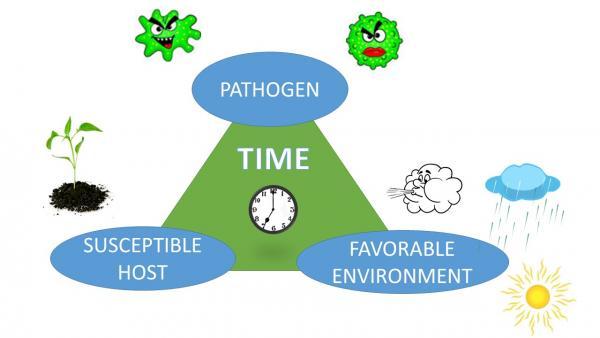
Figure 5–57. The disease triangle represents the fact that disease occurs only when a pathogen, a susceptible host, and a favorable environment are all present together, and a sufficient period of time has elapsed.
Spread and Survival of Pathogens
Another important consideration in disease control is how pathogens move around and how they survive when there is no host plant available. Some examples were mentioned above in the section on pathogen groups. Fungi, especially in the form of spores, can move via wind, water, insects, and human activity. Some species of fungi are so versatile that they produce different kinds of spores that may disperse in different ways. Bacterial cells are very often water-splashed from diseased to healthy plants. There they may colonize plant surfaces without immediately causing an infection. Working among plants while they are wet is a good way to spread both fungi and bacteria. Viruses often rely on insect or mite vectors for dispersal and for entry into a plant. Nematodes and their eggs move wherever the soil is moved.
It is critical to understand where and how pathogens overwinter. Many pathogens survive in plant parts that remain alive over the winter, such as roots, bulbs, stems, and buds. Soilborne diseases such as southern bacterial wilt survive in the garden soil. Once a given piece of ground is infested with the causal bacterium, known as Ralstonia solanacearum, it will remain infested indefinitely. Tomatoes planted there will succumb to the bacteria as soon as hot weather arrives. On the other hand, the occurrence of the Tomato spotted wilt virus is a game of chance each year, depending on the survival of the virus in weeds and the movement of the thrips vectors. At the furthest extreme are diseases such as downy mildew of cucurbits. This pathogen can overwinter only in warm climates like Florida’s. The spores are blown long distances on wind currents, reaching North Carolina in late May or June each year. The disease can arrive earlier if spores hitchhike a ride on infected transplants.
The Diagnostic Process
Before rushing in with a hasty diagnosis and recommending action to correct a plant problem, we must identify the cause. Some plant diseases are easily recognizable based on a specimen or photograph, but others may be difficult to identify. We recommend a structured diagnostic process. As you work through the steps below, be aware that a definitive diagnosis is not always possible or even necessary. In some cases, you will simply narrow the list of possibilities to the point where an informed management decision can be made.
- Step 1: Gather as much information as you can and take notes. You may want to use the clinic sample submission form as a guide. Also think of the “three Ws (where, when, what) and H (how)” of good reporting. Where is the problem occurring? Be sure to confirm that the plant was actually growing in your county. Plants brought from elsewhere could have problems that are uncommon in your area. In what setting is the plant growing, such as in a pot, in the ground, in a raised bed, or on a north-facing slope? When did the problem first occur? Has it progressed quickly or slowly? Has it spread? When were the plants sown or transplanted to their current site? When was the sample collected? What kind of plant is affected? Is just one species, cultivar or variety affected, or are there many affected? What kinds of plants were grown in this location last year? How was the plant managed in terms of water, mulch, compost, fertilizer, lime, irrigation, and pesticides? How was the weather in the weeks before the problem appeared?
- Step 2: Observe the specimen or photos from the site or both. First, verify that the plant has been identified correctly. Then look at the entire plant: roots, stems, leaves, flowers, and seedpods (if any exists). Does it seem to be the right size and color for a plant of the reported age? Do you notice any of the other symptoms listed in Table 5–1a – f? If so, do they occur in the upper portion of the plant, the lower portion, or toward the middle? Are the symptoms or signs different on the upper surface of the leaf versus the lower? If there are color changes, are they generalized across the foliage, or are they mainly between or along the veins? If there are leaf spots, what are their size and shape? If the plant appears wilted, was it that way in the garden? Or did wilting occur since the plant was dug or pulled? How does the leaf look when held up to the light? Are any parts of the plant missing? Look for signs of insects or mites. Their damage is sometimes mistaken for disease. Have leaves or flowers dropped off? What is the distribution of affected plants: scattered, grouped, or otherwise distributed? With turf problems, note carefully both the symptoms on the individual grass plants and whether the “stand symptoms” consist of small spots, larger patches, rings, or just scattered plants. See the Turffiles glossary for more information. In all cases, see if there are any signs of the causal organism, such as those listed in Table 5–2. Be aware that many fungi are secondary and grow on plant parts damaged or killed by another pathogen.
- Step 3: Compare your observations with the easily recognized diseases and disorders described in Table 5–3a – f and come up with a diagnostic hypothesis. Be aware that some symptoms are unique to particular diseases, whereas other symptoms can be caused by a wide range of factors. Wildly excessive thorn production on roses is a foolproof indicator of the Rose rosette virus, but not every rose-rosette-infected bush shows this symptom. Mosaics (Figure 5–11) are usually the result of a viral infection, as are ring spots (Figure 5–12). The famous exception to this is that African violets will develop ring spots when cold water contacts the leaves. Leaf spots with an angular or linear shape because the spots stop abruptly at the major veins often indicate a bacterial infection, downy mildew, or foliar nematodes. Wilting, on the other hand, can have many causes. See the special section “When Plants are Wilted.”
- Step 4: Double-check your diagnosis. Once you have a shortlist of possible causes, look for photos and descriptions in Extension publications or other references you may have on hand or online. Is there anything that can help rule out one or more of the possibilities? Make more observations if needed. With experience, you will be able to confidently identify a number of diseases. If you cannot reach any conclusions, show the sample to an Extension agent or the PDIC.
- Step 5: Make recommendations, if possible, based on what you were able to determine from the suggestions presented above and consult available extension publications for recommended ways of managing the problem. Sometimes there is nothing that can be done to alleviate a problem in a home garden situation. In other cases, cultural practices can reduce the impact of the disease this year or in the future. In a few cases, fungicide applications may be worthwhile. Always encourage integrated pest management (IPM) approach. A study of plant pathogens and the disease development and spread (will give you a better understanding of how diseases occur and which management strategies are helpful and when management is useless.
Be especially careful about the following situations:
Vegetables
- Necrotic spots or blotches on the leaves of crucifers (including cabbage, collards, and broccoli). These spots may be the result of black rot, caused by the bacterium Xanthomonas campestris. The typical v-shaped lesions are not always present, making diagnosis difficult at times.This disease has a lot of destructive potential.
- Wilting of tomato plants in the garden without a visible sign of fungal activity. (See When Plants are Wilted.) Whether the cause is nematodes, southern bacterial wilt, the early stages of southern stem blight, or something else entirely, wilting can have serious long-term implications for the garden.
- Leaf spots on tomatoes and peppers. Spots caused by bacteria, fungi, or even Tomato spotted wilt virus can look surprisingly similar. Some are more serious than others, and control measures are quite different.
- Large areas of tomato or potato foliage turning gray or gray-green and withering in a short period of time, often accompanied by large rotted spots on tomato fruit. These symptoms can indicate late blight, a very destructive disease that the PDIC helps to monitor. The timing of its appearance in North Carolina varies from year to year.
- Stem rots, leaf spots, and blights of cucurbits. Downy mildew, several true fungi, and two kinds of bacteria can cause leaf spotting in cucurbits. In addition, downy mildew is subject to monitoring because it shows up at different times and in different places each year. When stems are involved, the laboratory can help to differentiate between the various possible causes. Note that gummosis (Figure 5–27) on stems is a response to a number of kinds of stress, not just the disease known as “gummy stem blight.”
- Anything involving leaf distortion or dwarfing. These symptoms can be caused by viruses, mites, or insects, or by chemical injury.
Fruit and Nut Trees
- Fruit and nut trees in the home orchard present particular challenges for diagnosis. Refer anything to the PDIC that does not fit the pattern of one of the diseases described in Table 5–3c.
Other Trees and Woody Ornamentals
- Table 5–3d mentions a number of diseases of woody ornamentals. When none of those seems to fit, the PDIC can be of assistance. It is important to get a good sample that includes sufficient roots in soil, plus the right parts of branches and foliage. It normally is not enough to have just detached leaves, or stems that are completely dead.
- If dieback symptoms are present, carefully cut away a strip of outer bark from an affected branch. If you find a very clear boundary between live and dead cambium (or inner bark), it is likely a canker disease
Herbaceous Ornamentals
- Root and stem rots—For confident diagnosis of root rots in bedding plants, a microscope and selective media are often needed. The results are important because they affect the choice of replacement plants. (See “When Plants are Wilted.”)
- Blights—When large areas of foliage die in a short period of time. even when lower stems, roots, rhizomes, and other plant parts look good, then a laboratory diagnosis is helpful.
Important: Problem names can vary, depending on the host plant and the plant part affected.
GENERAL
Problem |
Occurs on |
Season* |
Hallmarks |
Cause |
Artillery fungus |
Leaves, walls, windows, cars |
(W, Sp) Su, F |
Circular, dark raised specks about one-tenth of an inch across. When scraped off, a persistent stained spot remains. |
Spore balls shot from the fungusSphareobolus growing on mulch. |
“Dog-vomit” slime mold (Figure 5–48) |
Mulch, walls, tree trunks, and other wood surfaces |
Su |
Yellow frothy masses several in. to a ft. wide, developing a pink crust over dusty dark spores. |
Fuligo septica, a harmless organism unrelated to fungi. |
Sooty mold |
Any plant |
Su, F |
Black mold on upper surface of leaf is easily wiped off with fingers (Figure 5–69). |
Fungi growing on insect honeydew. Not damaging to plants except blocking photosynthesis. |
Stinkhorns |
Mulched beds |
Su, F |
Foul-smelling, horn-shaped fungi with dark sticky tops and tan or orange stalks, developing from egg-like structures in the ground. |
Several fungi that develop in decaying plant material. (Some differ from the description at left.) |
*Time of year encountered in field and garden:. Sp—Spring, Su—Summer, F—Fall, W—Winter.
VEGETABLES
Problem |
Occurs on |
Season |
Hallmarks |
Cause |
Blossom-end rot |
Tomato, pepper |
Su |
Flattened dead areas on blossom-ends of fruit (Figure 5–52). Tan-colored, becoming dark (Figure 5–54) if secondary fungi invade. In pepper (Figure 5–53), can occur on the sides of the fruit. |
Calcium deficiency in developing fruit due to lack of Ca in soil or moisture fluctuations. |
Common smut |
Sweet corn |
Su |
Ears or other parts develop whitish galls that fill with dusty black spores. |
Ustilago maydis (fungus). |
Glyphosate injury |
Tomato is very sensitive, but any plant can be affected. |
Su, F |
In tomato: bright yellow coloration of youngest leaves, often at the base of leaflets. At higher doses plant death occurs. |
The drift of spray droplets from the nearby application of glyphosate herbicide. |
Powdery mildew |
Cucurbits (Figure 5–70), others |
Su, F |
White to gray fungal growth on upper and/or lower leaf surfaces. |
Different fungi, each specific to a group of plants, e.g.,Podosphaera xanthii on cucurbits. |
Root-knot nematode |
Cucumber (Figure 5–26), tomato, beet, okra, spinach, others |
Sp, Su, F |
Swellings are not easily detached from roots. Size variable. |
Nematodes in the genusMeloidogyne. |
Rust |
Sweet corn |
Su, F |
Small pustules break through the leaf surface, producing dry spores that are brick-red or orange. |
Two fungi in the genusPuccinia cause common and southern rusts. |
**Southern stem blight,
Southern blight,
Southern stem rot |
Many species (not corn) |
Su |
Rot on lower stems and other plant parts in contact with soil. In moist weather, a felt-like fan of white fungal growth on the stem (Figure 5–71). Round sclerotia (Figure 5–39) are white at first, turning into tan to brown “bb’s.”. |
Sclerotium rolfsii (fungus) |
**White mold,
Sclerotinia blight,
Sclerotinia rot,
“Drop” (lettuce) |
Crucifers, lettuce, sometimes others |
F, W, Sp |
Usually light-brown rot of stems and leaves (Figure 5–72). Fluffy white fungal growth. Irregular black sclerotia—similar in shape and color to mouse droppings—eventually form on or within stems. |
Sclerotinia sclerotiorum(fungus) |
**Until and unless fungal structures develop, stem rots are difficult to distinguish from one another.
FRUITS (TREE AND SMALL)
Note: A wide variety of problems can occur on fruits, and some resemble each other (for example, copper injury, bacterial leaf spot, and scab on peach fruit). Anything beyond the easily recognized diseases on this list should be referred to an experienced agent or to the PDIC. For more information, including recommendations on preventive sprays, see Disease and Insect Management in the Home Orchard.
Problem |
Occurs on |
Season |
Hallmarks |
Cause |
Bitter rot |
Apple |
F |
Circular rotten spots have a vee-shaped profile when the apple is sliced. Cream-colored to orange spores may exude from tiny dark fruiting bodies. |
Two species of the fungus Colletotrichum |
Black knot |
Stone fruits, esp. plum and cherry |
All |
Segments of twigs, branches, or trunks become swollen, black, and roughened (Figure 5–73). |
Apiosporina morbosa (fungus) |
Brown rot (Figure 5–20) |
Stone fruits |
Su |
Smooth, brown rotted areas expand rapidly as fruit mature. Fuzzy gray or tan fungal sporulation develops on the surface. |
Species of the fungus Monilinia |
Cedar-apple rust |
Apple |
Late Sp. to Su |
Bright yellow and orange leaf spots (Figure 5–74). Small yellow blisters on the upper leaf surface turn dark. Later, spore-producing pustules with frilled edges form on leaf undersides (Figure 5–42). (Use a hand lens to confirm.) |
Gymnosporangium juniperi-virginianae |
Flyspeck |
Apple |
Su, F |
Dark flecks, each the size of a period, in clusters on the surface of developing and mature fruit. |
Schizothyrium pomi (fungus) |
Gray mold
Also called botrytis blight on many plants |
Strawberry |
Sp |
Fuzzy gray mold on rotting fruit(Figure 5–75). Caution: This can be confused with several other problems if the mold is not yet present. |
Botrytis cinerea (fungus)
Diagnostic key |
Leaf curl |
Stone fruits |
Sp to early Su |
Expanding leaves become twisted and ruffled, often with reddening (Figure 5–76). |
Taphrina deformans (fungus) |
Mummy berry |
Blueberry |
Su |
Ripening berries turn the cream pink (Figure 5–21) instead of blue. They fall from the plant and eventually shrivel into a dry pumpkin-shaped “mummy.” |
Monilinia vaccinii-corymbosi(fungus).
More info on blueberry fruit diseases. |
Powdery mildew |
Many species |
Sp, Su, F |
White to gray fungal growth on upper and/or lower leaf surfaces. Can also occur on fruit (e.g., peaches, grapes). |
Multiple fungi, esp. Podosphaera(rosaceous hosts) and Uncinula(grape) |
Sooty blotch |
Apple |
Su, F |
Superficial, irregular dark areas on the skin of developing and mature fruit. |
Various fungi |
TREES AND SHRUBS
Problem |
Occurs on |
Season |
Hallmarks |
Cause |
Black spot |
Rose |
Sp, Su, F |
Dark leaf spots with “feathered” borders. Also yellowing and leaf drop (Figure 5–77). |
Diplocarpon rosae (fungus) |
Boxwood blight
(If suspected, send to PDIC for confirmation.) |
All boxwood species; also sweetbox and pachysandra |
All |
On boxwood: Dark-brown leaf spots, dark streaks on green stems, extensive leaf drop starting at the bottom of the plant. On pachysandra, leaf spot. |
Calonectria pseudonaviculata(fungus) |
Camellia petal blight |
Camellia japonica |
Sp |
Petals turn brown and blossoms drop off. When the base of the flower is removed, a ring of white fungal growth is visible on petal bases. |
Ciborinia camelliae (fungus) |
Cedar-apple rust |
Apple, crabapple |
Su |
On these hosts, mostly affects leaves. See section on fruit diseases, above. |
Gymnosporangium juniperi-virginianae (fungus) |
Cedar-apple rust |
Eastern redcedar |
Sp |
Woody, round galls on branches sprout orange gelatinous telial horns in mid-spring after warm rains. Comparison with quince rust (Figure 5–78). |
Gymnosporangium juniperi-virginianae (fungus) |
Cold injury |
Gardenia (Figure 5–79), pittosporum (Figure 5–80), boxwood (Figure 5–81), others |
All |
Not the same as frost damage to foliage or blooms. Typically, bark loosens or splits, often near the soil line. Dieback and decline may go unnoticed until the shrub is stressed by summer heat. |
Extremely low temperatures in late fall or early spring, when stems are not completely dormant |
Exobasidium leaf gall |
Camellia (Figure 5–24), azalea (Figure 5–82), rhododendron |
Sp |
Thickening or bulbous growths on new leaves. Whitish-green at first; may darken over time. |
Species of Exobasidium (fungus) |
Entomosporium leaf spot |
Red-tip photinia, Indian hawthorn (Figure 5–83), sometimes pear |
All |
Bright red leaf spots, whose centers turn gray over time and develop tiny blisters where spores are produced. Leaf drop can be severe. |
Diplocarpon mespili (fungus) |
Lichens |
All trees and shrubs |
All |
Flat (Figure 5–84), flaky (Figure 5–85), or hairy (Figure 5–86) growths on the bark of branches and trunks (also on rocks). Typically gray to grayish-green in color. |
Lichens are symbioses between algae and fungi. They’re often seen on declining plants, but do not cause direct damage. |
Normal leaf drop |
Southern magnolia, American holly (Figure 5–87), pines |
Sp, Su, F |
Pines, as well as broadleaved evergreens, shed their 2+-year-old leaves or needles. The time of year depends on the tree species. |
Natural aging. No cause for concern unless leaves formed during the last two years are dropping. |
Oak leaf blister |
All oaks |
Sp, Su |
Yellow-green to white puckered spots on newly expanded leaves. Spots turn brown in early summer. |
Taphrina caerulescens (fungus) |
Powdery mildew |
Euonymus (Figure 5–37), rose (Figure 5–88), saucer magnolia, dogwood, Leucothoë, spiraea, tulip poplar, many others |
Sp, Su, F |
White fungal growth on leaf and stem surfaces range from thin to felt-like. Dogwoods in midsummer get brown blotches on leaves (Figure 5–89) (hold edgewise to see the fungus). Leucothoë develops red leaf spots were infected (Figure 5–90). |
Multiple fungi, esp. Erysiphe andPodosphaera |
Quince rust |
Eastern redcedar (Figure 5–91) |
Sp |
Needles or slight branch swellings develop small orange gelatinous telia in early spring. Comparison with cedar-apple rust (Quince rust on eastern red cedar (Figure 5–78). |
Gymnosporangium clavipes(fungus) |
Quince rust |
Ornamental pear (Figure 5–92) |
Su |
Pustules (aecia) of orange spores develop on fruit or swollen twigs. Tubular white membranes over the aecia are obvious on fruit. |
Gymnosporangium clavipes(fungus) |
Shot-hole |
Cherry-laurel and flowering cherry |
Su, F
(All, if on ever-green species) |
Leaf spots turn from green to brown and drop out, leaving holes with clean edges (Figure 5–8). |
Several fungi and bacteria |
Slime flux |
White oak, elm, other hardwoods |
Su |
In the heat of summer, fermented sap oozes from cracks in bark (Figure 5–93). On white oak, this is usually close to the soil line. The sour odor attracts a variety of insects (Figure 5–94). |
Anaerobic bacteria cause a condition called wet wood. Gas from fermentation forces sap out |
Spot anthracnose
(not the same disease as dogwood anthracnose) |
Flowering dogwood |
Sp |
Small red or tan spots develop on showy bracts (Figure 5–95). Bract can be deformed if spots are numerous. |
Elsinoë corni (fungus) |
HERBACEOUS ORNAMENTALS (ANNUAL AND PERENNIAL)
Problem |
Occurs on |
Season |
Hallmarks |
Cause |
Dodder |
Many hosts |
Su, F |
Masses of thin, orange strands grow among and attach to stems of the host plant (Figure 5–21). Inconspicuous white flowers may be present. |
Parasitic plants in the genusCuscuta |
†Impatiens downy mildew |
Standard impatiens and its hybrids (not New Guinea) |
Su |
Leaves yellow and drop (Figure 5–96), leaving only stems that eventually rot. Velvet layer of white spores (Figure 5–97) develops on the underside of leaves in humid weather. |
Plasmopara obducens(fungus-like organism) |
Leaf streak |
Daylily |
Su |
Leaves turn brown from the tip (Figure 5–98). The leading edge of the dead tissue is in the form of a narrow “V” along the midrib. |
Aureobasidium microstictum(fungus) |
‡Phytophthora aerial blight |
Madagascar periwinkle (annual vinca) |
Su |
Leaves on individual stems wilt and die, turning gray-green and brown. Leaves do not stick together. Often there are distinct brown or purple lesions on stems (Figure 5–99). |
Phytophthora nicotianae(fungus-like water-mold) |
Powdery mildew |
Coreopsis, gerbera, monarda, sedum, zinnia, and others |
Sp, Su, F |
White to gray fungal growth on upper and/or lower leaf surfaces (Figure 5–100). |
Several different fungi, each specific to a group of plants |
Root-knot nematode |
Begonia, impatiens, liriope, others |
Sp, Su, F |
Swellings are not easily detached from roots. Galls tend to be large and numerous in begonia and impatiens (Figure 5–101) but can be small (e.g. in liriope). |
Nematodes in the genusMeloidogyne |
Rust |
Aster, daylily, hollyhock, Jack-in-the-pulpit, oxalis, St. John’s-wort, others |
Su, F |
Yellow or orange spots on the upper leaf surface (Figure 5–102). Pustules of yellow to orange to reddish brown, dry (often dusty) spores on the underside (Figure 5–103) of each spot. |
Many fungi includingPuccinia, Uromyces, andColeosporium; specific to their hosts |
†A similar disease affects basil and cucurbits, but we recommend diagnosis at the PDIC when there are foliar problems in those plants.
When Plants Are Wilted
A plant can wilt (droop) during all or part of the day. This is often accompanied by a loss of luster in the green color. When wilting occurs within individual leaves, you may see large tan-colored dead areas between the veins, sometimes extending to entire leaves.
Several problems can lead to wilting. It may be an indication of the following:
- The plant lacks sufficient water in the root zone, either because of a lack of rain or irrigation, excessive drainage, or hydrophobic (water-repellent) soil.
- Heat and low humidity are causing a plant to transpire moisture more quickly than it is able to pull water from the soil.
- Excess soluble salts in the soil—usually because of excess fertilizer applications—are limiting water uptake.
- Decay has affected roots or lower stems. Root rot often starts in wet weather, but the wilting may not be apparent until hot, dry weather sets in. At that point, there are not enough healthy roots left to supply the plant’s water requirements.
- Root-feeding nematodes have compromised the root system. One common group of nematodes causes visible swellings on roots, but many kinds do not. Nematode feeding may or may not be accompanied by noticeable root decay.
- A vascular disease such as southern bacterial wilt or fusarium wilt is interfering with water transport. In these cases, the xylem vessels in the roots or stems are unable to move water up to the leaves, fruits, and other plant parts.
- Wilting caused by insect attacks or infectious diseases often starts with just a few plants or even one side of a plant. If most or all plants of a certain kind are affected in a short period of time, it is likely a cultural or environmental issue.
When an herbaceous plant has wilted in the vegetable garden or flower bed in spite of adequate soil moisture in the root zone, the following schematic can be used. It is set up in the format of a “dichotomous key.” Starting at the top, decide whether statement 1a or statement 1b better applies to the situation. Depending on the answer, you will either be directed to a diagnosis or to another set of numbered comparisons. Repeat the process until you reach a diagnosis or recommendation.
1a. Evidence of insect tunneling in stem (insect, webbing, frass, shed skin) insect injury; see chapter 4, “Insects”
1b. No sign of insects feeding in or on stems
2a. Visible decay at the base of the main stem, near the soil line (also check roots)
2b. No visible decay at the base of the main stem
3a. Visible fan of white fungal mycelium (Figure 5–35) and/or small, round, tan sclerotia (Figure 5–39) southern stem blight
3b. Little or no evidence of fungal growth unidentified stem rot;
4a. Roots darkened, decaying, or missing root rot,
4b. Good number of roots present, neither darkened nor decayed
5a. Areas of roots swollen into knots that do not easily detach root-knot nematode
5b. No swellings on roots vascular wilt, nematodes, or excess fertilizer; submit a sample
Note: This text refers to symptoms seen in herbaceous plants in the garden or flower bed. Trees and shrubs can respond differently to the kinds of stresses listed here.
|
†Normal roots of some plants are naturally dark. A good test for root rot is to tug on a root to see whether the outer portion can be easily pulled off of the central core of vascular tissue.
‡Root knots are reliably large in some plants such as tomato, cucumber, impatiens, and begonia. They may be small and hard to see in some kinds of plants, in which case a clinic diagnosis will be needed.
Principles of Plant Disease Management
Although homeowners and growers are sometimes simply curious to know the cause of a particular disease, most are interested in controlling it. Often no acceptable measures are available that will halt the disease, and the affected plant or plants must be removed. Sometimes the only thing that can be done is to redouble preventive efforts for the next growing season. In other cases, the affected plant will recover by putting out new roots and leaves with or without the application of management measures. Diagnosis of the disease is very important in deciding whether the problem is serious and what measures, if any, will be helpful and cost-effective. The choice of management measures is influenced by where the pathogen overwinters, how it spreads, its host range, and how the conditions favoring infection. See the previous sections on Disease Development and Spread and Survival of Pathogens.
In many cases, it is not realistic to talk about disease control. We do not have complete control over nature, even in our own backyards. Thus, the sections that follow will discuss the two main types of disease management strategies: cultural and chemical. Many homeowners and growers today are interested in biological methods of dealing with pests. Unfortunately, there are very few biological options for plant disease management in the yard and garden at the present time. At the end of the chapter, we will pull these ideas together within the framework of integrated pest management using a case study model.
Cultural Management
We often hear these sayings about managing problems: “A stitch in time saves nine.” Or “An ounce of prevention is worth a pound of cure.” Taken literally, this means that what we do to prevent disease is 9 to 16 times more effective than dealing with a disease after it has become established. Many of these preventive measures fall under the category of cultural practices, the actions we take every day as part of growing plants. A summary of the top practices for disease management in the garden is given in the highlighted box. Notice that all but one are cultural methods.
Follow the “right-plant-right-place” approach. Before planting, carefully study the site. Does it have the appropriate amount of sun exposure, drainage, and soil for a chosen plant to thrive? Boxwoods and dogwoods, for instance, prefer light shade. Although they may appear to tolerate full sun, it predisposes them to problems. Azaleas and daphne need good drainage to avoid root rot. |
Besides adaptability to your soils and climate, look for plants that are not susceptible to common diseases. Even within susceptible species, look for varieties and hybrids that offer resistance to key diseases. For example, there are apple cultivars that are resistant to cedar-apple rust, dogwood cultivars resistant to powdery mildew, rose cultivars resistant to black spot and cercospora leaf spot, and tomato cultivars resistant to nematodes and some fungal wilts. Heirloom tomato varieties have become increasingly popular but may lack resistance to soilborne diseases. In this case, grafting is an option. For more information, see the Grafting for Disease Resistance in Heirloom Tomatoes by Cary Rivard and Frank Louws (AG-675), and keep an eye out for a grafting workshop near you.
Plant with an eye toward diversity. If all the plants in the vicinity are of the same type, a disease can spread quickly. Diversified plantings are less likely to suffer major disease problems. You could, for instance, use groups of different shrubs for a screen rather than just Leyland cypress or Japanese cedar.
Rotate crops to try to break the disease cycle. Change the location in the garden where crops are planted from one year to the next. Vegetable crops in the same family, such as cabbage and broccoli, should not follow each other in the same spot in the garden. The length of an effective rotation will depend on the disease involved, so get a diagnosis. Some pathogens, unfortunately, do not disappear over time. An example is Ralstonia solanacearum, the cause of southern bacterial wilt. Once introduced it will remain in the soil indefinitely.
Improving the soil will reduce plant stress and decrease soilborne diseases. Good soil drainage, proper soil pH, and optimum fertility produce healthy plants that are more resistant to insects, diseases, and environmental stresses. Soil testing will reveal pH and nutritional problems that can reduce growth and lead to plant stress. Learn more about soil testing in chapter 1, Soils and Plant Nutrients. Planting in beds raised 6 to 8 inches above grade and adding organic amendments to the soil can significantly reduce problems with phytophthora root rot.
Buy seed from a reputable source, and inspect all transplants, including the roots, before planting. Boxwood blight finds its way into landscapes in North Carolina by the installation of new, infected plants.
Among plant pathogens, most nematodes, many fungi, some bacteria, and a few viruses are soil inhabitants. Take care not to move soil among landscapes or gardens on borrowed or rented tools or equipment. For the same reason, transplanting shrubs or perennials from one location to another risk transplanting pathogens as well as the plant.
Plant and harvest at recommended times, to give every advantage to the plant. For example, Madagascar periwinkle (Catharanthus roseus) should not be planted until the soil has warmed in the spring. Planting when the soil is cold and wet can also cause okra seeds to rot in the ground. While late plantings of squash are very likely to suffer from powdery mildew, pansies should not be transplanted until the soil temperature has cooled in the fall.
Because water is the major limitation for bacteria and most fungi, be careful with irrigation. Overwatering favors water mold species like Phytophthora andPythium and the root rots they cause. Avoid wetting leaves and stems if possible to prevent foliar diseases. If overhead watering cannot be avoided, at least water during times that will not add hours to the natural nighttime leaf wetness period. Space and prune plants properly to improve air circulation and help the foliage dry quickly.
In some cases, prompt removal of diseased plants or plant parts can reduce the amount of a pathogen in the vicinity. For example, the removal of diseased tomato leaves can slow the progress of septoria leaf spots. If a tree or shrub in a hedge planting has been diagnosed with armillaria root rot, the individuals on either side should also be removed, because they have likely been infected via fungal growth through the soil. The same strategy would be of little benefit with Phytophthora root rot because the spores of Phytophthora species can move far beyond the initial infection in water runoff.
Remove plant debris in the fall to reduce pathogen populations. Many fungal foliar pathogens overwinter in dead leaf litter. In the case of fallen camellia blossoms, rake them up in the spring. Sclerotia of the camellia petal blight fungus, Ciborinia camelliae, survive the whole year on the ground’s surface after the blossoms rot away. The sclerotia resume growth and release spores in the spring, to coincide with the emergence of camellia blossoms. In the vegetable garden, remove roots if they were infested with nematodes or root rot pathogens. Liriope (Liriope muscari) should be trimmed close to the ground in late winter to reduce the carryover of anthracnose on foliage from one year to the next. Fire blight, brown rot, and many other fruit diseases overwinter in cankers. Even the fungus that causes black spots on roses can overwinter on canes as well as fallen leaves. Prune and remove cankers during the dormant season by removing 4 to 6 inches into clean wood. When pruning out active fireblight infections in the spring, be more aggressive, going 10 to 12 inches below the visible damage. Destroy the clippings, or at least remove them from the site, and sanitize shears frequently. See the NC State Extension sanitizers table for more information.
When composting, turn the pile thoroughly so it reaches a high enough temperature to kill pathogenic fungi, bacteria, and nematodes. Even though compost pile temperatures might get high enough to kill pathogens, you should never knowingly place infected plant material in the compost pile. Read more about composting in chapter 2.
If pathogens are present, cultivate flower beds and vegetable gardens. Cultivation can expose soil insects and disease organisms to desiccation, cold temperatures, and predation.
A 2-to-3-inch layer of mulch acts as a barrier between the soil and the plant and may prevent inoculum-infested soil from splashing onto the plants during watering or heavy rain. Mulching will also enhance the total health and vigor of most plants. Late winter mulching can reduce camellia petal blight and the early infections of black spots on roses. In both cases, the mulch covers overwintering fungal structures so that spores are not released into the air.
Many pathogens take advantage of wounds to enter, so avoid any unnecessary injury. This is especially important with trees, where roots are often disturbed by digging, and the consequences may take years to develop.
Remove weeds while they are young. Some weeds can harbor diseases and arthropod vectors. Mow or destroy weeds from ditch banks and other nearby areas. Till the planting area early, so weeds that might carry pests have time to die before you plant.
A physical barrier can protect certain plants. Tomatoes, peppers, and eggplants benefit from this technique; they can be protected from cutworms and the soilborne disease southern blight. Wrap the stem of transplants with a 4-by-4-inch strip of aluminum foil so that 2 inches of the stem is protected above and below the ground.
A solid planting of French marigolds (Tagetes patula) will give good control of many kinds of root-knot nematodes. Details of the practice can be found in the NCDA&CS publication NemaNote 1. A common misconception is that a sprinkling of marigolds can discourage nematodes. In fact, planting only a few marigold plants in a vegetable garden will provide little or no control.
Solarization is an approach that involves heat-treating the soil using energy from the sun to reduce populations of nematodes, weeds, and soilborne fungi. It has potential benefits in approximately the eastern half of North Carolina. The soil should be covered with plastic for at least four weeks, preferably longer, during a hot and sunny time of the year. Frequent cooling by rain and cloudy periods will reduce the benefits.
To achieve maximum results, follow these recommendations:
- Till the soil well before covering it with plastic to destroy clods and plant debris, which might interfere with uniform conduction of heat through the soil and protect some organisms from the full effects of the treatment.
- Make sure the soil is moist when you apply the plastic cover. Wet soil conducts heat better than dry soil. Also, most pest organisms are more susceptible to the lethal effects of heat in moist soil; they may be dormant if dry.
- Raise the center of the bed so that it forms a small crown or peak to enable the plastic to shed water. Water standing on the plastic will absorb some of the heat intended for the soil.
- Use clear plastic instead of black plastic. Clear plastic produces higher temperatures faster than black plastic.
- Use thin plastic (1 to 2 millimeters), which permits more sunlight to penetrate to the soil and has been reported to affect more rapid and deeper control for soilborne fungi than thicker plastic (6 millimeters).
- Leave the plastic cover on until planting time. It has no detrimental effects on the soil and will reduce the chances of recontamination before planting.
- Avoid bringing contaminated plants or untreated soil into the treated bed when planting, and do not till or otherwise disturb treated soil. Deep tillage can bring soil up from depths that were not adequately heated.
Chemical Management
Prevention and sanitation are the most practical approaches to disease control and have been detailed in the previous section. Home gardeners have few alternatives for chemically controlling plant diseases, and almost all of those alternatives are directed against fungi. Although the products used are called fungicides, they slow down rather than eliminate fungi. There are few, if any, chemical options for consumers to use against bacteria, nematodes, and viruses.
Fungicides are often unnecessary because many diseases cause only minor aesthetic damage. In other cases, fungicides may be impractical to apply. They are of no value against cankers or root rots in trees and shrubs. However, fungicides can be an important component of IPM in some situations. It is impossible to control black spots on susceptible rose cultivars without fungicides. A homeowner with a peach or apple tree will need a high tolerance for damaged fruit if he or she chooses not to spray. Fungicides can also be important tools in the management of certain turfgrass diseases.
No single fungicide will control all fungi, though some have a wider spectrum of activity than others. Most fungicides are protectants and should be applied before a pathogen infects a plant. Furthermore, many do not move within the plant and so must be applied repeatedly to protect new growth. Some fungicides are systemic and can cure early infections.
For a fungicide to be a useful tool, the disease must be properly diagnosed and the right chemical must be applied in a timely manner, at the correct dose, with good coverage, and at the necessary intervals. |
Do not expect miracles from fungicides. For example, fungicides applied after powdery mildew is present on a leaf will not return the leaf to its original green color. A fungicide can, however, protect new growth from becoming infected.
Fungicide Classification
Fungicides can be classified by their topical mode of action (how they interact with the plant) or their biochemical mode of action (how they affect the target fungus).
There are four basic topical modes of action.
- Contact fungicides. These are strictly protectants and must be on the surface of the plant before infection takes place. They break down and wash off over time and so must be reapplied fairly frequently, usually every 7 to 10 days. Reapplication is also necessary because new growth is not protected.
- Translaminar fungicides (local penetrants). These compounds will move into and across a leaf but not up or down in the plant.
- Acropetal penetrants. These fungicides will enter the plant and move from the point of entry in an upward direction only, with the transpiration stream.
- True systemics. Only one group of fungicides—the phosphonates—will move downward from the point of application. One implication is clear: For most fungicides, if you want activity in the crown or roots, you have to apply to the crown or roots!
There are dozens of biochemical modes of action, but they can be conveniently grouped into two groups: “multisite” and “single-site” modes of action. Fungicides classified as having “multisite” modes of action are all broad-spectrum protectants with a contact topical mode of action. These chemicals disrupt a wide array of life processes in the fungal cell. On the other hand, fungicides with “singlesite” modes of action affect very specific biochemical processes in the fungal cell. They usually have a narrower spectrum of activity and are often—but not always—local or acropetal penetrants. One limiting factor in using these compounds is that some fungal populations can evolve to be less sensitive, or have a resistance to a compound after repeated use (see below).
Recent years have shown increased interest in chemicals that have fungicidal activity but are not fungicides in the traditional sense. Examples in this category include petroleum-based horticultural oils, neem oil (derived from the neem tree), and potassium bicarbonate (a relative of baking soda, used primarily against powdery mildew). When used against certain diseases, these chemicals can be adequate alternatives to conventional fungicides when the disease pressure is not too great. Keep in mind that neem is toxic to bees.
The repeated use of pesticides can lead to the evolution of insensitive pest populations, whether they are fungi, bacteria, insects, weeds, or rodents. In such cases, individual pests are not becoming stronger. Instead, chance mutations result in resistant individuals, which are the ones that survive and reproduce. Whether and how fast resistance becomes widespread enough in a population to result in loss of control depends on the biochemical mode of action of the pesticide, the life history of the pest, its reproductive rate, and the intensity of pesticide use.
Fungicide Timing
The timing of fungicide applications is an important component in chemical disease control. Fungicides should be applied when conditions favoring disease development are present.
Rainy, foggy, warm, and humid weather conditions generally favor disease development. Whenever possible, spray schedules should be adjusted to provide fungicide protection before rainy periods, and fungicides should be reapplied after heavy rains if the label so indicates.
When using chemical management, we need to know the life cycle of the disease organism and when it is most susceptible. Many fruit diseases are best managed when the fruit is very young, or even in the blossom stage; once the fruit starts to rot, it is too late. A diagnosis at that time can at least help a gardener plan management strategies for the next season.
Always carefully read and follow the label when using any pesticide.